Supplementary methods: A streamlined CRISPR pipeline to reliably generate zebrafish frameshifting alleles
Jared C. Talbot and Sharon L. Amacher
Check our website (http://molgen.osu.edu/amacher/resources) for the most up-to-date version of the protocol.
We welcome comments and discussion at our blog (http://zCRISPR.blogspot.com).
- Table of Contents 1
- Identify CRISPR targets
- Get to know the target gene 2
- Identify a target region 4
- Identify a target site 5
- Design primers for HRMA and sequencing 6
- Example of target site design: six1a 7
- Prepare templates for guide RNA (gRNA) synthesis
- Option 1: Cloning free method to generate gRNA
- Design guide-oligos 8
- PCR amplify guide-oligos 9
- Gel extract PCR-amplified guide-oligo 9
- Option 2: Plasmid based method to generate gRNA
- Design and order insert-oligos 10
- Generate BsaI-digested pDR274 fragment 10
- Clone insert-oligos into pDR274 11 o Prepare targeting plasmid for gRNA synthesis 11
- Option 1: Cloning free method to generate gRNA
- Generate gRNA and cas9 mRNA
- Synthesize and clean up gRNA 12
- Prepare linear pCS2-nCas9n plasmid 13
- Prepare nls-zCas9-nls mRNA 14
- Mutagenize embryos (generation “F0” ), and test mutagenesis efficacy
- Co-inject zebrafish embryos with gRNA and nls-zCas9-nls mRNA 15
- Lyse embryos for HRMA 15
- Introduction to High Resolution Melt Analysis (HRMA) 16
- Set up high resolution melt reactions 17
- Analyze F0 melt curves 18
- Recover and sequence the first generation of outcrossed founders (F1)
- Screen founders 19
- HRMA instructions for F1 screening 20
- Prepare heterozygous DNA for sequencing 21
- Analyze sequences from heterozygous DNA 22
- Example of allele selection: choosing between three six1a alleles 23
- Stock maintenance in subsequent generations 23
- Confirm lesions in second generation (F2) homozygotes
- Identify adult F1 founders 24
- Identify homozygous mutants in F2 24
- Sequence the homozygous mutation 25
- Example of sequence analysis on six1aoz9 heterozygotes and homozygotes 25
- Validating the CRISPR pipeline 26
- Supplies and equipment 27
- Brief bibliography 28
II ) Identify CRISPR targets
Get to know the target gene:
Before beginning CRISPR design, itʼs important to have a good understanding of the gene you intend to mutate (the “target gene”). To begin, check the literature for existing phenotypic, expression, and domain information about the target gene:
- Check ZFIN (www.zfin.org) and the Mouse Genome Informatics (MGI) website (http://www.informatics.jax.org/) for expression patterns, existing mutants, and publications.
- Look for overlapping expression of orthologous genes in ZFIN and MGI. If there is a related protein with overlapping expression, consider targeting the ortholog as well.
- In particular look for duplicate genes resulting from the teleost genome duplication, because these duplicated gene pairs (paralogs) often function in a partially redundant fashion with one another. Known paralogs are typically named in the format ʻgene1aʼ and ʻgene1bʼ. Even if a target geneʼs paralog is not explicitly listed, you may find one by blasting (see below). If present, strongly consider generating double mutants of both paralogs (gene1a;gene1b).
- Check Pubmed for published functional analysis on your gene of interest.
- In particular, search for information that reveals functionally important portions of the target gene and/or protein.
Next, assemble a set of annotated files for the target geneʼs genomic, protein, and mRNA sequences:
- Obtain genomic sequence, with exon structure annotated.
- Check both Ensembl and NCBI, because sequences sometimes differ in the two databases. If major differences are found, compare genomic structure of the genes with homologues from other species to identify the best zebrafish sequence.
- To export annotated genomic sequence from NCBI, search for the target and species of interest in the “Gene” category. Open the desired gene page, and under the “genomic regions, transcripts, and products” section click the “genbank” link. To export this sequence, copy the text from “LOCUS” to “//”, and paste into a text file. This exported file can be imported into viewers capable of reading GenBank files, such as Geneious (proprietary) or SnapGene viewer (freeware).
- To export annotated genomic sequence from Ensembl, search for the target gene and click the link to the chromosomal location. Click “export data”, and change the format to “GenBank”. Various features can be selected or deselected according to taste, but make sure that at minimum “Vega Gene Information” and “Variation Features” tracks are selected. Click “Next”, and then click “text”. Exported sequence can be pasted into a text file, and imported into viewers.
- The genomic sequences exported from Ensembl contain annotations of alternative splice forms. Make note of exons that are sometimes skipped, particularly if exon skipping is shown in Vega annotations.
- Look for alternative splicing in other species, even if those exons are not shown to have alternative splicing in zebrafish. Multi-species comparisons of exon structure can also be done using the UCSC genome browser (https://genome.ucsc.edu/) by searching for the human version of the target gene, and using the “configure” button to show the “vertebrate chain/net”. Sequences can be exported from this browser using the “View” then “DNA” buttons. If alternative exon usage is found in some species, note this on the zebrafish sequences.
- Obtain protein sequences from a variety of species, and use these to identify conserved features of the target gene.
- Protein sequence files can be exported from Ensembl or NCBI, similar to genomic sequence files. In NCBI, these sequences can be found under the “mRNA and Protein” section of the gene page.
- In addition, annotated protein files can be directly imported to Geneious software using the “NCBI” search function. Simply search for your gene name and Danio, identify the correct sequence, and copy-paste the file into your desired folder.
- Blast the human and zebrafish protein sequences against the zebrafish genome to search for hidden duplicates and orthologs. You can BLAST sequences using several databases, including www.ensembl.org,!blast.ncbi.nlm.nih.gov, and www.phylogeny.fr.
- Check both Ensemble and NCBI for protein domain annotations. o In the absence of published data, protein domains can also be identified using bioinformatics tools such as Pfam ( http://pfam.xfam.org/ ).
- Align protein sequence using freeware (e.g. http://www.ebi.ac.uk/Tools/msa/clustalo/ ) or proprietary software (e.g. Geneious). For these alignments, we typically use sequences from zebrafish (including duplicates if present), at least one other fish, a Xenopus species, chicken, mouse, and human.
- Examine alignments of orthologous proteins to identify more putative domains, which may be annotated on proteins from other species.
- Annotate strongly conserved regions of the zebrafish protein, even if they do not contain known domains.
- Obtain mRNA sequence, and annotate with information about genomic and protein structure.
- mRNA sequence with 5ʼ and 3ʼ untranslated regions annotated can be obtained in a manner similar to protein sequences.
- Annotate the mRNA file with exon boundaries identified above, and highlight exons that are sometimes skipped.
- Annotate the mRNA file with sequences encoding conserved protein features and domains.
- This annotated mRNA file should now contain information about genomic sequences (exon structure), mature splice form of the mRNA, and the protein that is encoded by the mRNA. It will be used to identify the ideal region for CRISPR mutagenesis.
Identify a target region
Once familiar with the target gene, it is important to hone in on the correct target region for mutagenesis. The target region is an exon, or portion of a large exon, found towards the 5ʼ end of the target gene that optimally satisfies the criteria set forth below. Use the mRNA file annotated in the previous section to learn which region of the target gene will most likely cause a strong loss of function. We use the following guidelines, written in order of importance, to help identify the best region.
- The target region should be 5ʼ to domains that you suspect may impart functionality.
- The target region should be 3ʼ to potential alternative start sites (in frame ATGs or even CTGs1 at the beginning of the gene). Alternative start sites can also be avoided by selecting a target region 3ʼ to short deeply conserved sequences at the 5ʼ end of a gene (e.g. signal peptides).
- Use RepeatMasker (http://www.repeatmasker.org/) to identify repeat sequences. These are usually rare in exons, but should be excluded from CRISPR target regions if present.
- The target region should be in a constitutive exon. Avoid exons that are sometimes excluded from transcripts unless your project focuses on function of a particular splice form.
- Preferred target regions are located on the 5ʼ end of exons containing at least part of a domain that appears important to protein function.
- Preferred exons have few or no SNPs shown in Ensemblʼs “Variation Features” track.
- Avoid choosing the first exon in poly-exonic genes, because of the prevalence2 of alternative first exon usage.
- Exons longer than 150 bp are likely to contain ideal CRISPR sites. If a shorter exon is chosen, be aware that short exons can be hot spots for alternative splicing.
- If you intend to use HRMA for CRISPR tests and mutant screening, it is important that the target region has uniform melting characteristics, without irregularities caused by local melting domains. If uMelt software (https://www.dna.utah.edu/umelt/um.php 3 ) predicts irregularities in a sequenceʼs melt curve, derivative curve, or melting profile (Red asterisks below), then trim the target region to one predicting uniform curves (preferably >150 bp).
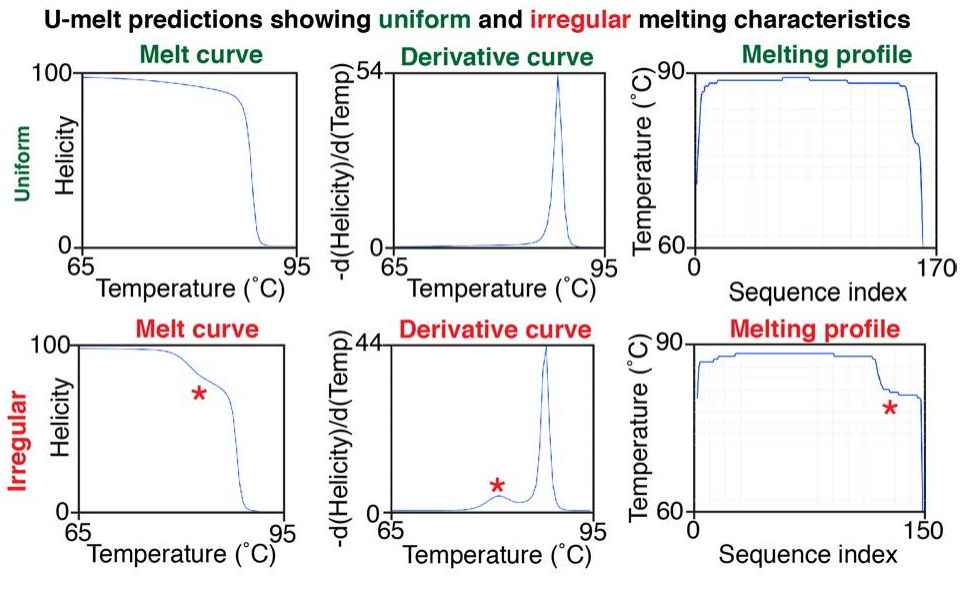
Identify a target site
There are several websites that design targets for CRISPR mutagenesis. We have mainly used ZiFiT software 4, 5 for design, so this section follows the ZiFiT workflow. Other CRISPR design websites, including “Mojo Hand” 6 (http://www.talendesign.org/), “CRISPR Design Tool” 7 (http://crispr.mit.edu/), and CHOPCHOP 8 (https://chopchop.rc.fas.harvard.edu/index.php), can also be used. It is especially helpful to check multiple sites if few or no target sites are found in the target region you have chosen based on the preceding criteria.
- Navigate to the ZiFiT website (http://zifit.partners.org/ 4, 5 ), and follow links to the “CRISPR/Cas nucleases” site.
- Copy/paste the target region from the target geneʼs genomic sequence file into the text box.
- Select “T7 Promoter” which will ensure that the CRISPR target begins with “GG”, the preferred starting bases for T7 RNA polymerase. This criterion can be relaxed if no hits are returned that satisfy the guidelines below.
- If a suitable target beginning with GG is not found, then de-select “T7 promoter”, and find a target preferably beginning with NG or GN; alter the sequence to read “GG” 9 (see example on page 7). Alternatively, the Sp6 promoter can be used, which lacks the GG requirement. A recent report indicates that the Sp6 promoter generates highly active gRNA 10.
- Specify the length of target site, typically 20 bp. Recent reports have shown that 17 bp or 18 bp target lengths work well, and may even improve mutagenesis specificity 11.
- Select “Zebrafish” for species.
- Click “Identify target sites”.
- Click “Identify potential off target sites”. Eliminate candidate targets that have off-target sites containing only 1 bp or 2 bp mismatches compared to the target. Ensure that the correct target (0 bp mismatch at the correct genomic location) is returned in this list of “off target” locations.
- Annotate a few non-overlapping targets with low predicted off-targeting on the mRNA sequence file.
- Eliminate candidate CRISPR target sites with an obvious stretches of self-complementary sequence that might cause hairpin formation.
- Prioritize candidate CRISPR targets that overlap a restriction enzyme site, since restriction analysis provides an alternative method to assay mutagenesis and genotype. Restriction sites can be identified using NEBcutter (http://tools.neb.com/NEBcutter2/ ) or other platforms. Alternatives to HRMA are particularly important when genotyping incrosses of heterozygotes (see Notes on page 24).
- Select the most 5ʼ preferred CRISPR target(s), and annotate onto genomic sequences.
- Annotate the Protospacer Adjacent Motif (PAM), which is the short sequence (NGG) following the CRISPR site where cleavage occurs.
- Generate HRMA primers to genomic sequences surrounding the most 5ʼ CRISPR target site (see page 6), and check that the amplicon sequence predicts a smooth melt curve using uMelt software.
- If the 5ʼ furthest candidate CRISPR target within the target region passes the above selection, then use it to make CRISPR gRNA for mutagenesis. If not, then assess other possible candidate targets in a 5ʼ to 3ʼ direction.
- In our experience, the majority (>60%) of genes we have targeted are effectively mutagenized using the first gRNA we construct. A second CRISPR gRNA targeting another site can provide a backup for mutagenesis, as well as an independent allele.
Design primers for HRMA and sequencing
Before designing a gRNA, ensure that you can design good primers flanking the CRISPR target site for HRMA and sequencing. Sequencing primers are rarely problematic, but we have needed to redesign several CRISPR targets that could not be paired with good primers for HRMA. We order HRMA and sequencing primers from Operon at 10 nMole scale, with salt-free cleanup. Primer design uses standard software (e.g. Primer3 http://bioinfo.ut.ee/primer3/). In addition, we suggest the following guidelines for reliable analysis.
HRMA primers:
- Check for predicted SNPs in the target region using the “variation features” track on Ensembl. If there are SNPs predicted in the PCR product, we recommend sequencing the amplicon from the fish you intend to inject. If SNPs are already segregating in the background, HRMA of CRISPR-injected fish will be complicated.
- The product size should be 100-160 bp; with these short PCR products, small sequence changes can produce large changes in melting temperature.
- Ensure that at least 15 bp are present between each primer and the CRISPR target site, so longer insertions or deletions (indels) can be detected.
- Test HRMA primers for predicted melt curves using “uMelt” software (https://www.dna.utah.edu/umelt/umelt.html 12 ). Using this software, ensure that the predicted PCR product gives a smooth melt curve, with only one melt peak. If multiple melt peaks are predicted on wild-type DNA, then analysis of mutant DNA will be difficult.
Sequencing primers:
- Ensure that at least 50 bp are present between each sequencing primer and the CRISPR target site.
- Ensure that at least one of the primers for sequencing is found in the same exon as the CRISPR target site. Intronic sequence and untranslated regions (UTRs) sometimes contain polymorphisms that insert or delete sequences, complicating lesion identification.
Example(of(CRISPR(target(design:(six1a
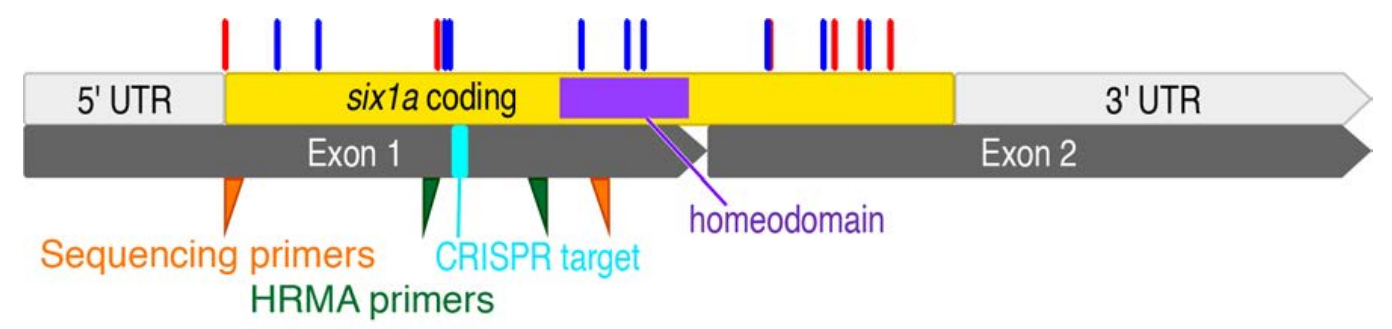
We uploaded and analyzed six1a genomic sequences from Vega (OTTDARG00000022888), and NCBI (Gene ID: 494168); the two databases showed perfect agreement so we only uploaded NCBI sequences for mRNA and protein files. six1a is a two exon (gray) gene. The protein encoded by six1a is strongly conserved across chordates, with >91.6% identity in pairwise comparisons between species (lamprey six1, Danio six1a, Danio six1b, X. laevis six1, chicken Six1, mouse Six1, human SIX1). Although we typically avoid targeting the first exon, we chose to target the first exon of six1a because this exon contains a deeply conserved homeodomain (purple). Ensembl prediced no SNPs in the coding sequence. To target downstream of any potential alternative start sites, we marked the transcript with in-frame AUGʼs (red) and CUGs (blue), and selected a target region between these sites and the homeodomain:
GAAGGCGCACTATGTTGAGGCGGAAAAGTTGCGGGGGCGCCCGCTAGGCGCTGTGGGCAAATATCGTGTGCGCAGGAAATTCCCTTTGCCCCGTACTATCTGG
Using ZiFit software, we identified target sites towards the 5ʼ end of this region. One target site (GGGGCGCCCGCTAGGCGCTG) worked well with a T7 promoter, but was likely to make hairpins, and caused low to no mutagenesis. A second, non-overlapping, target site (aqua) was selected at the 5ʼ end of the target region:
Target site [PAM]: GAAGGCGCACTATGTTGAGG[CGG]
This target is incompatible with T7 promoter, so we modified it to accommodate the promoter (GgAGGCGCACTATGTTGAGG). Alternatively, we could have left the target unchanged, and used an Sp6 promoter instead of T7.
We designed HRMA primers (six1a_HRM_F: GCACAACCACCCGAAGATG and six1a_HRM_R: CTCGCCGTCCCAGATAGTAC) (green triangles) to amplify sequences around this six1a target. These primers predict a 145 bp product and a smooth melt curve using uMelt software which we confirmed using HRMA. We also designed sequencing primers (six1a_Seq_F: ATGTCAATCTTGCCCTCGTTCG and six1a_Seq_R: TGGATAAGGGTTGTGCGTGTAC) (orange triangles) that amplify within the first exon of six1a and produce a 447 bp product centered near the CRISPR target site. We ordered short oligos to clone this CRISPR target into pDR274 (see page 10), and used the resulting plasmid to generate gRNA for six1a mutagenesis. We also describe an alternative, cloning-free, method to generate gRNAs (see page 8) that we now use routinely.
IIIa) Cloning-free method to generate gRNA
Based closely on protocols described in Hruscha et al 13. Templates for guide-oligos can be generated using either this cloning-free method, or a cloning-based method described on pages 10- 11. The cloning-free method requires a more expensive oligo (119 bp), but saves labor, reagents, time and sequencing compared to the plasmid-based method. By our calculations, these savings compensate for the cost of 119 bp oligos.
Design guide-oligos
“Guide-oligos” are synthetic DNA strands, which are used to generate templates for guide RNA synthesis. Guide-oligos are made of three main parts: a T7 promoter, a variable targeting sequence, and a Cas9 binding scaffold. A short clamp is provided to stabilize the 5ʼ end of double-stranded sequence. The full sequence of a generic guide-oligo is shown below:
GCGTAATACGACTCACTATAGGNNNNNNNNNNNNNNNNNNGTTTTAGAGCTAGAAATAGCAAGTTAAAATAAGGCTAGTCCGTTATCAACTTGAAAAAGTGGCACCGAGTCGGTGCTTT
To design a guide-oligo, simply replace the underlined region in the above sequence with your target sequence. T7 transcribes the last base (G) of its promoter, and also prefers a G in the next position. These two Gs are included in the target sequences identified by ZiFiT software, when the “T7 promoter” option is selected. The underlined example above is shown with 18 Nʼs, plus the invariant 5ʼ GG for a 20 bp target, but target lengths may vary. To design gRNA with an Sp6 promoter, replace the green sequence with “ATTTAGGTGACACTATA”, and then add targeting sequence without the GG restriction 10.
ZiFiT outputs the targeting sequence in the correct orientation, but double-check that the sequence begins with GG. As another check, the CRISPR binding site ends just before the PAM sequence (NGG) following the target. This end should “point” towards the gRNAʼs Cas9 binding scaffold sequence, such that the PAM sequence will coincide with the terminal “GTT” of the scaffold sequence. If you plan to transcribe mRNA directly from this synthesized guide-oligo, be sure to reverse-complement your design. For consistency with previous publication 13, we purchase guideoligos in the reverse-complemented orientation, but we transcribe from a PCR-amplified product instead of directly transcribing from the guide-oligo (see page 9).
Guide-oligos can be ordered as long primers, without special purification. We order guide-oligos, typically 119 bp long, from Operon at the 0.2 µM scale with salt-free cleanup. Oligos of this length can also be purchased from other vendors, such as Sigma or IDT.
Example: guide-oligo for six1a
Page 5 describes target site design. Target[PAM]: GgAGGCGCACTATGTTGAGG[CGG]
Insert target sequence into the guide-oligo template sequence: GCGTAATACGACTCACTATAGgAGGCGCACTATGTTGAGGGTTTTAGAGCTAGAAATAGCAAGTTAAAATAAGGCTAGTCCGTTATCAACTTGAAAAAGTGGCACCGAGTCGGTGCTTT
Reverse complement to generate guide-oligo sequence (order this): AAAGCACCGACTCGGTGCCACTTTTTCAAGTTGATAACGGACTAGCCTTATTTTAACTTGCTATTTCTAGCTCTAAAACCCTCAACATAGTGCGCCTcCTATAGTGAGTCGTATTACGC
PCR amplify guide-oligo
Because guide-oligos are lengthy, the oligo synthesis received from manufacturer contains a complex mixture of nucleotides and isnʼt pure enough to use directly as templates for mRNA synthesis. A simple PCR efficiently amplifies the 119 bp guide-oligo sequence, yielding the desired product.
___Resuspend guide-oligo in low TE (10 mM Tris-HCl, 0.1 mM EDTA), to generate a 100 µM stock. Store this stock at -20˚C.
___Dilute this stock to 1 µM in sterile H2O
___Prepare PCR mix:
___10 µl 10 X Thermopol buffer
___2 µl 10 mM dNTP
___2.5 µl 10 µM gRNA_Primer1/2
___1.5 µl Taq (NEB M0267S)
___2µl 1 µM guide-oligo
___79 µl Sterile H2O.
___3 µl DMSO
gRNA Primer 1: GCGTAATACGACTCACTATAG
gRNA primer 2: AAAGCACCGACTCGGTGCCAC
___Split PCR into two 50 µl wells.
___Run PCR: 95˚C 1 min (95˚C 15 sec, 60˚C 30 sec, 72˚C 20 sec) 40X, 72˚C 5 mins, 4˚C ∞
___ Run PCR product on a 3% agarose gel in 1x TBE at 180 volts for ~1 hour. Load 1µl on a narrow well for imaging, and the remaining 99 µl on a wide well for extraction. Expect a single bright band at 120 bp.
Gel extract PCR amplified guide-oligo
___Gel extract PCR product, using a Qiagen MinElute gel extraction kit (Qiagen 28604), or another gel extraction kit.
___Setup vacuum manifold (Qiagen 19413) with MinElute column and manifold adapters (Qiagen 19407).
___Cut out the 120 bp band from the 99 µl lane.
___Place in a 15 ml pre-weighed conical tube.
___Re-weigh the conical.
___Calculate the necessary volumes for isopropanol (same as gel volume), and Buffer QG (six times gel volume), as described in kit instructions.
___Add 6 gel volumes Buffer QG to the samples.
___Place at 50˚C for 15 minutes. Vortex occasionally.
___Add 1 gel volume isopropanol; mix by tilting a few times.
___Transfer to MinElute column, 700 µl at a time. Apply vacuum.
___Wash with 500 µl Buffer QG. Apply vacuum.
___Wash with 750 µl Buffer PE (apply Buffer PE to the columnʼs lip to rinse away Buffer QG). Apply vacuum.
___Wash with 750 µl Buffer PE a second time. Apply vacuum.
___Place in collection tube, and spin 1 minute “empty” in a tabletop centrifuge
___Transfer columns to nuclease-free microfuge tubes.
___Add 12 µl H2O (nuclease-free).
___Let sit 1 to 2 minutes.
___Spin 1 minute.
___Quantify sample via spectrophotometry (yield should be ~10-50 ng/µl). Donʼt be alarmed by low readings. The product is so short that 30 ng of template has higher molar concentration than 1 µg of a typical plasmid.
IIIb) Plasmid-based method to generate gRNA
This method is based closely on protocols described in Hwang et al 14. This plasmid-based method is more laborious than the cloning-free method, but creates an archivable plasmid. Both the plasmidbased and cloning free methods can be used to generate high quality gRNA.
Design and order insert-oligos
___Follow instructions above (page 5) to identify a target site.
___From the ZiFiT design website, copy/paste the “oligo 1” and “oligo 2” sequences for this target into an excel spreadsheet. These are the insert-oligos.
___If using a different design program, the insert-oligos can be designed using the target site. To generate “insert-oligo 1”, simply add “TA” 5ʼ to the target sequence. To generate “insert-oligo 2”, delete the GG at the beginning of the target sequence, then reverse complement the target sequence, and finally add “AAAC” 5ʼ this sequence. When mixed together, the insert-oligos will create a double-stranded targeting sequence flanked by 4 bp single-stranded overhangs, compatible with the overhangs generated by BsaI digestion of pDR274 (see below).
___Order the insert-oligos as standard primers with salt-free cleanup.
Example: making insert-oligos to target six1a
Page 7 describes target site (GgAGGCGCACTATGTTGAGG) design.
insert-oligo 1 is: TAGgAGGCGCACTATGTTGAGG
insert-oligo 2 is: AAACCCTCAACATAGTGCGCCT
Generate BsaI digested pDR274 fragment
___Obtain pDR274 from AddGene (plasmid #42250).
___Streak bacterial stock carrying pDR274 onto LB plates (10 g/L Tryptone, 5 g/L yeast extract, 10 g/L NaCl, 15 g/L agar; pH 7.5) containing 50 µg/ml Kanamycin.
___Pick isolated colonies, and start an overnight culture in LB broth (10 g/L Tryptone, 5 g/L yeast extract, 10 g/L NaCl; pH 7.5) containing 50 µg/ml Kanamycin.
___Miniprep pDR274.
___Linearize pDR274 with BsaI-HF (New England Biolabs R3535S):
___10 µg plasmid
___10 µl BsaI-HF
___10 µl CutSmart Buffer
___ Sterile H2O to 100 µl
___Place at 37˚C for at least three hours.
___Purify linearized plasmid using QiaQuick PCR cleanup kit (Qiagen 28104). Use two columns to extract the sample, because each can purify up to 5 µg. Elute using 30 µl H2O (nuclease-free) per column.
___Run 1 µl digested and undigested plasmid on a 1% agarose gel for diagnosis. Expect to see a single band at 2.1 kb
Once prepared, a stock of linearized pDR274 can be used for many CRISPR cloning reactions.
Clone insert-oligos into pDR274
___Resuspend insert-oligos to create a 100 µM (1000x) stock solution of each oligo in Low TE (10 mM Tris-HCl, 0.1 mM EDTA).
___Mix 10 µl insert-oligo 1 and 10 µl insert-oligo 2 1000x oligo stocks in 80 µl Low TE to generate a 10 µM (100x) stock of the insert-oligo pair.
___Mix 10 µl of the 10 µM stock in 990 µl Sterile H2O for 0.1 µM insert-oligo pair working stock.
___In an 8-well strip, mix together:
___1 µl linearized pDR274
___1 µl T4 ligase (New England Biolabs M0202S, 400 U/µl)
___2 µl 10x T4 ligase buffer
___2 µl 0.1 µM insert-oligo pair working stock
___14 µl H2O (nuclease-free)
___Place in PCR machine, water bath, or incubator, and run at 16˚C for 3 hours to overnight.
___Thaw competent bacteria, having a transformation efficiency of at least 108 colony forming units per microgram, on ice.
___Dispense competent bacteria into three 25 µl aliquots in chilled microfuge tubes:
___Add 5 µl of ligation to one tube
___Add 5 µl of Sterile H2O to a second tube
___Add 1 µl of Kanamycin-resistant positive control plasmid (10 pg/µl) to the third tube
___Leave on ice for 15-30 minutes.
___Heat shock at 42˚C for 30 seconds.
___Add 125 µl SOC media (20g/L tryptone, 5g/L yeast extract, 10 mM NaCL, 10 mM MgCl2, 10 mM MgSO4, 20 mM glucose).
___Shake the cells for one hour at 37˚C.
___Plate 100 µl of ligation or H2O control by spreading onto LB plates with 50 µg/ml Kanamycin. Only plate 1 µl of control plasmid.
___Let liquid absorb a few minutes, then place plates at 37˚C overnight.
___Score transformations in the morning. When using competent cells with an advertised transformation efficiency of 109 colony forming units per microgram, we typically find 10-300 colonies per ligation plate, no colonies on the H2O control plate, and 50-300 colonies on the positive control plate.
___Start two 3 ml overnight cultures per ligation, in LB containing 50 µg/ml Kanamycin. Grow overnight at 37˚C while shaking or rotating.
___The next morning, create a frozen bacterial stock. Mix 750 µl of the overnight culture with 250 µl of 80% sterile glycerol in a cryo-safe screw cap vial, and store this stock at -80˚C.
___Use the remaining culture to prepare plasmid DNA (miniprep).
___Sequence-confirm plasmids using M13F sequencing primer. Select a clone that carries the correct insert; this is the targeting plasmid.
Prepare targeting plasmid for gRNA synthesis
___Digest the targeting plasmid using DraI (New England Biolabs R0129S):
___5 µg plasmid
___4 µl DraI
___5 µl CutSmart Buffer
___Sterile H2O to 50 µl
___Place digestion mixture at 37˚C for at least three hours.
___Run digests on a 1% agarose gel. Expect to see bands near 286 bp and 1.9 kb.
___Gel extract the 286 bp fragment as described on page 9.
IV ) Generate guide RNA (gRNA) and cas9 mRNA
Synthesize and clean up gRNA
Prepare reaction mixture using templates generated on page 9 or page 11, and components provided by the MAXIscript T7 kit (Life Tech AM1312, or AM1310 for Sp6). Use RNAse-free technique.
___Thaw dNTPs and templates on ice. Thaw TXN buffer at 40-50˚C. Keep T7 enzyme and RNAse inhibitor on ice.
___Mix together at room temperature:
___ >30 ng template PCR product or linearized targeting plasmid
___1 µl ATP
___1 µl UTP
___1 µl CTP
___1 µl GTP
___2 µl 10X TXN buffer
___2 µl T7 enzyme
___1 µl RNAse inhibitor (Promega N2515)
___ H2O (nuclease-free) to 20 µl
___Place reaction at 37˚C for 3 hours to overnight.
___Add 1 µl Turbo DNAse.
___Incubate at 37˚C for 15 minutes.
___Add 2 µl 8M LiCl (nuclease-free, Sigma L7026) (see Note 1).
___Add 75 µl of pre-chilled 100% EtOH (nuclease-free. Fisher BP2818).
___Place at -80˚C for 1 hour to overnight.
___Spin 15 minutes at 4˚C.
___Wash with 200 µl pre-chilled 80% EtOH (nuclease-free).
___Spin 5 minutes at 4˚C.
___Remove all liquid.
___Let pellet dry 5-10 minutes.
___Resuspend in 30 µl H2O (nuclease-free).
___Quantify sample via spectrophotometry (concentration should be ~300-600 ng/µl) (See note 2).
___Run on a 3% agarose diagnostic gel (see Note 3):
___Pre-heat PCR machine to 72˚C.
___Mix 3 µl RNA, 2 µl H2O (nuclease-free), 5 µl molecular biology grade formamide in an 8- well strip.
___Place strip in 72˚C PCR machine for 3-5 minutes.
___Load hot samples onto a 3% agarose gel, alongside 5 µl MassRuler DNA ladder (Fisher Fersm0403).
___Run gel 20-30 minutes at 160 V. Expect to a band below 80 bp, around 120 bp, and sometimes a faint “laddering” above 120 bp (see Note 4).
___Store immediately at -80˚C.
Notes
- LiCl precipitation is less expensive than column-based methods, is simpler, is non-toxic, and in our experience gives better yields than column-based cleanup for small products.
- When synthesis is successful, spectrophotometry readings correlate well with band intensities. However on some occasions, synthesis fails entirely, revealed by poor band intensity on a gel despite high spectrophotometry readings. Synthesis is not considered successful unless bright bands of appropriate size are seen on a gel, even if spectrophotometry readings are high.
- Although less precise than an acrylamide gel, this diagnostic gel works well and is considerably simpler to prepare.
- The extra bands may represent different folding structures, or annealed RNA.
Prepare linear pCS2-nCas9n plasmid
___Streak pCS2-nCas9n 15 (Addgene 47929) frozen stock onto LB plates containing 50 µg/ml Carbenicillin or 100 µg/ml Ampicillin.
___Pick isolated colonies, and start 3 ml overnight culture in LB plus 50 µg/ml Carbenicillin or Ampicillin. Avoid picking satellite colonies that will lack the plasmid, particularly if Ampicillin is used as a selective agent instead of Carbenicillin.
___Perform a Qiagen midiprep of the pCS2-nCas9n plasmid.
___Linearize pCS2-nCas9n with NotI-HF (NEB R3189S):
___20 µg plasmid
___10 µl NotI-HF
___10 µl CutSmart Buffer
___Sterile H2O to 100 µl
___Place at 37˚C for at least three hours.
___Purify linearized plasmid using QiaQuick PCR cleanup kit (Qiagen 28104). Extract using two QiaQuick columns, which each have 10 µg capacity, and elute into 30 µl H2O (nuclease-free) per column.
___Run 1 µl digested and undigested plasmid on a 1% agarose gel for diagnosis. Expect a single band at 8.2 kb in the digested sample.
Synthesize nls-zCas9-nls mRNA
___Thaw 2X NTP-CAP and linearized pCS2-nCas9n on ice. Thaw 10X Sp6 buffer at 37-50˚C. Keep Sp6 enzyme and RNAse inhibitor on ice (see Notes below).
___Assemble reaction mixture at room temperature:
___0.5-2 µg (up to 7 µl) linearized pCS2-nCas9n 15
___2 µl Sp6
___2 µl 10X Sp6 buffer
___10 µl 2X NTP-CAP
___1 µl RNAse inhibitor (Promega N2515)
___H2O (nuclease-free) to 20 µl
___Place reaction mixture at 37˚C for 4 hours.
___Add 1 µl Turbo DNAse.
___Place at 37˚C for 15 minutes.
___Add 80 µl H2O (nuclease-free).
___Pre-mix 300 µl Buffer RA1 with 300 µl 100% EtOH (nuclease-free).
___Add 600 µl RA1/EtOH mixture (from previous step) to sample. Pipette to mix.
___Transfer to Nucleospin RNA II columns.
___Spin 30 sec. Discard flow through.
___Add 350 µl Buffer MDB. Spin 1 min. Discard flow through.
___Add 200 µl Buffer RAW2. Spin 30 sec. Discard flow through.
___Add 600 µl Buffer RA3. Spin 30 sec. Discard flow through.
___Add 250 µl Buffer RA3. Spin 30 sec. Discard flow through.
___Spin column empty for 2 minutes.
___Transfer columns to new microfuge tubes.
___Add 40 µl H2O (nuclease-free), and let sit 1-2 minutes.
___Centrifuge for 1 minute; flow-through is the nls-zCas9-nls product.
___Quantify product by spectroscopy. Concentration should be 300-800 ng/µl.
___Run 2 µl on a 1% agarose diagnostic gel. High quality nls-zCas9-nls mRNA shows prominent bands around 800, 1000, and 1500 nt, that may represent different folding structures.
___Immediately store RNA at -80˚C in 800 ng aliquots.
Notes on nls-zCas9-nls mRNA synthesis
- We prefer to use a nuclear localized version of cas9 that is optimized for codon usage in zebrafish (nls-zCas9-nls), which is easy to synthesize and shows high activity in zebrafish embryos 15.
- Use RNAse-free technique throughout this protocol.
- Use mMessage mMachine Sp6 kit (Life Tech AM1340) for synthesis, and “Nucleospin II RNA cleanup kit” (Machery Nagel 740955.50) to purify the RNA; the reagents listed above, including proprietary buffers RA1, MDB, RAW2, and RA3, come from these kits.
- LiCl purification of nls-zCas9-nls is an inexpensive alternative to column purification. For LiCl purification, follow directions on page 12, but give extra time for the final pellet to dissolve in nuclease-free H2O. With a limited sample size, LiCl purification of nls-zCas9-nls works as well as purification using the Nucleospin kit.
- Syntheses of nls-zCas9-nls showing high spectrophotometry readings, but low band intensity on gel, are considered failed, and should be discarded.
- When synthesizing 4.2 kb nls-zCas9n-nls mRNA, we do not use the MAXIscript kit recommended for gRNA synthesis, because the MAXIscript kit does not add a 5ʼ cap and is not recommended for transcripts larger than 2 kb.
V ) Mutagenize F0 embryos, and test mutagenesis efficiency
Co-inject zebrafish embryos with gRNA and nls-zCas9-nls mRNA
___Prepare injection mixture on ice on the morning of injection:
___ gRNA to 40 ng/µl final concentration
___ nls-zCas9-nls mRNA to 80 ng/µl final concentration
___2 µl 1M KCl
___0.5 µl 0.5% Phenol Red
___H2O (nuclease-free) to 10 µl
___Inject 1-2 nanoliters of injection mixture directly into the blastomere of early one-cell stage embryos. To ensure injection into this early stage, collect batches of embryos every 10-20 minutes and inject no more than 40 eggs per collection.
___Transfer embryos to 28.5˚C incubator.
___Clean embryos a few hours after injection. Note numbers of healthy, deformed, and infertile embryos.
Lyse embryos
___Dechorionate 8 uninjected and 8 injected embryos at one to three days post fertilization.
___Transfer embryos to columns one and two of a 96 well plate. To ensure uniform volumes, embryos can be transferred using a p20 set to 2.5 µl and fitted with a p200 pipette tip. Using scissors or a razor, cut 2-3 mm off the end of this p200 tip, to accommodate the width of an embryo.
___Add 20 µl 1x Thermopol buffer (New England Biolabs, B9004S).
___Place in PCR machine. Run 95˚C 10 minutes, 4˚C ∞.
___Add 5 µl 10 mg/ml Proteinase K to each well.
___Run 55˚C 1 hour, 95˚C 10 minutes, 4˚C ∞.
___Mix samples in each well.
Introduction to High Resolution Melt Analysis (HRMA)
The melt curve of a PCR product can be revealed using dyes which only bind to double-stranded DNA. Each DNA sequence produces a characteristic melt curve, which can be discerned from one another even when the sequences being compared differ by a single base-pair. This feature of DNA melt curves allow researchers to quickly identify heterozygous sequences in the progeny of CRISPR founders. Using HRMA, researchers can also quickly assess whether a target sequence has been mutagenized, which would result in a mixed WT/mutant population of PCR products with different melt characteristics than purely WT PCR products. Unlike most other means of mutant discovery, such as surveyor assays, HRMA has the ability to quickly distinguish two different mutants from one another without sequencing. HRMA requires researchers to use several curves which plot DNA fluorescence as a function of temperature or amplification cycle; below we offer explanations and guidelines for interpreting these curves. See also Dahlem et al. 12 for use of HRMA to detect zebrafish mutations.
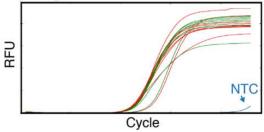
Amplification curve
The amplification curve shows the amount of DNA fluorescence in "Relative Fluorescence Units" (RFU) at each cycle of amplification. Any amplification of no template controls (NTCs) should be at least ten cycles later than wells with templates. Fluorescence increases as a function of double-stranded DNA quantity.
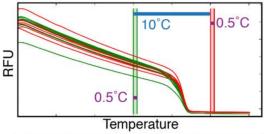
Melt Curve
The melt curve is a plot of fluorescence intensity vs temperature. Sample amplification differences make it difficult to determine melt differences from this graph.
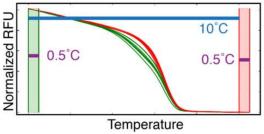
Normalized melt curve
The normalized melt curve allows melt profiles to be analyzed independent of amplification level. Normalized melt curves are generated by setting normalization borders before (green bar) and after (red bar) the peak melting temperature.
For consistency, we set the normalization borders 10oC apart, with widths of 0.5oC.
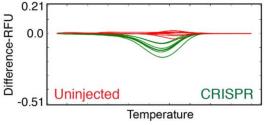
Difference Curve
The difference curve sets a reference group as a constant RFU of "0" and allows easy visualizatoin of how and experimental group differs from that reference (measured in "Difference-RFU).
Difference curves highlight small changes in melt temperatures, and also provide a metric for melt deflections to be quantitatively compared between experiments.
Expect most CRISPR injections to cause a downward deflection, because heteroduplex DNA typically melts at a lower temperature than WT (Homoduplex) DNA.
All of our CRISPR injections so far have caused deflections between 0.21 and -0.51. We scale our difference curves to this range, so the relative mutagenesis of different CRISPRs can be comprared. Injections that cause deflections of at least -0.1 difference-RFU reslut in a high founder rate.
Set up High Resolution Melt Reactions
Based on techniques described in Dahlem et al 12.
___Prepare HRMA mix.
Recipe for 16 samples:
___180 µl Precision Melt Mix (BioRad 172-5112)
___9 µl 10 µM HRMA primer pair (see Note 1)
___171 µl Sterile H2O
___Vortex
Scalable recipe (total volume = V):
___1/2 V Precision Melt Mix
___1/40 V 10 µM HRMA primer pair
___19/40 V Sterile H2O
___Vortex
___Transfer 18 µl HRMA mix to all 16 wells of Columns 1 and 2, plus wells 3A and 3B, of a 96 well plate (Bio-Rad; MLL9601) (see Note 2).
___Add 2 µl of embryo lysis (from Section VI) to wells of Columns 1 and 2 (see Notes 3 and 4).
___Add 2 µl sterile H2O to wells 3A and 3B (“no template” controls).
___Cover plate tightly with a transparent seal (Bio-Rad; MSB1001), using the corner of a lab marker to press the seal into the gaps between wells.
___Vortex briefly to mix, and then briefly microfuge the plate.
___Place the plate in an HRMA-capable qPCR machine.
___Run the following program to generate amplification and melt data:
95˚C 3 mins, (95˚C 15 sec, 60˚C† 20 sec, 70˚C 20 sec*) 45x,
65˚C 30 sec, melt 65-95˚C § , 95˚C 15 sec.
† Annealing temperature varies, but 60˚C works well for most primers.
* After 70˚C extension, image plate to generate amplification data.
§ During melt steps, change temperature 0.2˚C per step, and hold each step for 5 seconds before imaging the plate, to generate the melt data.
___Analyze amplification and melt curves using instructions on next page.
___If HRMA confirms CRISPR activity, raise the remaining embryos.
Reaction assembly notes
- HRMA primer design instructions are found on page 6.
- When adding HRMA Mix to wells, use “positive pressure pipetting” to ensure uniform sample distribution. When pulling liquid from the master mix, compress the pipettorʼs plunger two stops, and suck up liquid through both the normal level and the blowout. When dispensing HRMA mix, expel only to the first plunger stop (no blow-out). Refill, while maintaining the blowout reserve and expel to the first plunger stop again. Repeat, until all wells are filled.
- Avoid bubbles when dispensing template; expel template only to the first plunger stop while pipettors are immersed in liquid. Then, drag the pipettor up the side of the plate while compressing the plunger to the second stop (blow-out).
- Small differences in buffering can cause notable shifts in melt curves, and buffering can even be affected by the type of biological samples used. For instance, HRMA of wild-type fin clip DNA samples cannot be directly compared with HRMA of wild-type embryo DNA samples. Consistent results are obtained by keeping conditions constant for all samples within an experiment. For instance, the relative shape of wild-type versus heterozygous samples will appear the same when assayed using DNA from either fins or embryos.
- Per manufacturerʼs instructions, once a tube of Precision Melt Mix has been thawed, the unused remainder can be stored at 4˚C and used up to several months later, but should never be re-frozen.
Analyze F0 generation melt curves
- The following steps are written for Precision Melt Analysis software on a CFX Connect machine from BioRad. Illuminaʼs ECO machine and software also worked well for us, although the details of analysis differ slightly. In addition free software for HRMA is available online (https://www.dna.utah.edu/uv/uanalyze.html 16 ); this software can produce normalized melt curves, but not difference curves.
- First, check the amplification curves using qPCR software. Exclude samples that did not amplify well. Samples with low amplification plateaus often show different melt curves from samples with high amplification plateaus, even if they are the same genotype. Be wary of samples with linear amplification, rather than sigmoidal amplification. “No template control” should not amplify, or should amplify at least ten cycles later than samples with DNA.
- Next, open a melt file in Precision Melt Analysis software.
- In this software, normalize the melt curves to a set scale. The degree of mutagenesis can be compared by plotting melt deflections on a “Difference curve”, with a normalized scale (see page 16). To do this, set the borders of melt deflection (X-axis) to a 10˚C range, centered on the mean melting point. Adjust the pre-melt and post-melt border widths to 0.5˚C each. Ensure that the lower border is set before melting begins in CRISPR injected embryos (a downwards bubble in the melt curves). Adjust the Difference-RFU scale (Y-axis) to encompass 0.21 to - 0.51 Difference-RFU. These borders ensure that CRISPRs with a wide range of activities can be compared with one another on the same scale.
- Mutagenesis creates heteroduplex DNA which lowers melting temperature, shifting melt curves of CRISPR-injected embryo away from the uninjected embryoʼs melt curves. Uninjected embryo melt curves should cluster tightly together, whereas successfully mutagenized CRISPR-injected embryos will produce a variety of melt deflections.
- In F0 embryos, mutagenic CRISPR activity should induce a melt deflection of -0.2 to -0.4 Difference-RFU. Melt deflections causing | 0.05 | Difference-RFU or less indicate poor CRISPR activity, and often are observed even among wild-type embryos.
- When exporting images of melt curves, generate a cleaner image by deselecting the Grid Line and Minor Tick checkboxes under curve chart options. Save the image as a jpeg file with a resolution of 300 dots per inch.
- Use melt curves to determine the number of F0 (injected) fish to raise. If CRISPR injection causes strong deflections (mean >0.2), then most injected fish will carry mutations in the germline, and a small stock of 25 injected fish will be sufficient for mutant recovery. If CRISPR injection causes weak deflections (mean ~0.07), then only a minority of injected fish will transmit germline mutations, and a larger stock of 50-100 injected fish is recommended for reliable mutant recovery. See page 26 for formulas to estimate mutation rates.
VI ) Recover and sequence F1 generation mutant carriers
Screen founders
We use HRMA to screen CRISPR-injected founders for germline transmission of potential mutants (putants). This analysis reveals which CRISPR-injected fish transmit putants, how many putants are found per founder, and which putant embryos are the best candidates for sequencing.
___Outcross CRISPR-injected F0 fish to a wild-type line such as AB.
___Collect and clean F1 embryos at a few hours post-fertilization, and raise at 28.5˚C.
___At 1 to 3 days post fertilization, dechorionate 16 embryos per founder and lyse these embryos as described on page 15.
___Similarly process a set of 8 wild-type embryos, which will provide a reference curve to distinguish new mutations from wild-type polymorphisms or variation within a single wild-type genotype; this is particularly important when screening strongly mutagenized CRISPR founders, which may produce few to no wild-type offspring.
___Set up HRMA reactions as described on page 17.
___Analyze the samples as shown on next page.
___Put away identified carriers in individual containers. Segregate, or sacrifice, F0 fish that are unlikely to carry mutations.
___Raise the remaining F1 embryos from identified founders.
___Once an ideal lesion is selected (page 22), use the rate of that lesion to calculate the total number of fish required to make a stock of at least 6 F1 carriers. For example, if 1/16 embryos shows the desired lesion, then raise at least 96 fish. Continue outcrossing the identified founder until you have raised this number of fish. With six mutant carriers in the F1 generation there is a 97% chance that at least one carrier of both genders will be recovered, which will allow for incross and preliminary phenotypic characterization. Raising a much larger stock is not necessary, because the F1 generation should only be used for preliminary phenotypic analysis and for producing F2 generation stocks.
___At least once during the subsequent outcrosses, perform HRMA on 16 embryos to confirm the germline transmission rate for the desired lesion.
HRMA instructions for screening F1 embryos
- Ensure that amplification occurs properly, as described on page 18.
- Open up the melt file in Precision Melt Analysis software.
- Once in melt analysis software, scale the graph based on all embryos from all founders (the “All Founders graph”). Identify a single embryo towards the center of the wild-type melt curves (the “Mean Wild-Type Sample”).
- Next, divide the plate into “groups” by founder family. Scale graphs for each family to match scaling in the All Founders graph. Include the Mean Wild-Type Sample in each group.
- Most heterozygous mutants (both insertions and deletions) will melt at a lower temperature than wild-type and show negative deflections. Be wary of difference curves that show many embryos with positive deflections (indicating a higher melting temperature than wild-type). This usually means that the wrong sample has been selected as the wild-type reference.
- For each founder family, specify the Mean Wild-Type Sample as a new cluster and set that cluster as the reference. This way, each founder family can be directly compared to one another and to the total set of founders.
- The Precision Melt Analysis software automatically clusters melt curve types together, but these clusters often need manual polishing. Look closely at the curves, and adjust the meltclusters as necessary.
- Wild-type and putant curves typically make smooth (if complex) lines on melt and difference curves. Jagged, irregular, curves are suspect and may represent problems in amplification; exclude these from further analysis.
- Be wary of melt clusters that show the same deflection shape in multiple founders, which may be background alleles. To check for melt clusters common to multiple founders, adjust the colors from the All-Founders graph to match those seen in single-family graphs; this manual color adjustment often separates distinct putants that would have otherwise appeared to cluster together.
- If HRMA clusters are ambiguous, we often run the HRMA PCR products (1µl of each sample) on a 3% agarose gel. Many indels are large enough to generate distinct band patterns that can be used to refine clustering.
- Each validated melt-cluster typically represents a shared lesion. Different melt clusters typically represent different lesions.
- Give each melt-cluster a name; these are your putants. We name putants by gene, then founder number, then a letter for each cluster. For instance, six1a CRISPR founder 7 gave rise to three putants: six1a-7a, six1a-7b, six1a-7c, the “putant A, putant B, and putant C” shown in Figure 1 of the accompanying article.
- Record the identity (putant name or wild-type) of each well.
- The best founders transmit at least two clear putants, at high rates. Raise offspring from these founders, and prioritize these putants for sequencing.
Prepare heterozygous DNA from F1 embryos for sequencing
Set up PCR reactions using the sequencing primers designed on page 6.
___Prepare master mix, using Phusion polymerase (New England Biolabs M0530L).
For 8 samples:
___ 80 µl Phusion HF buffer
___ 12 µl DMSO
___ 10 µl sequencing primer pair (10 µM each)
___ 8 µl dNTP mix (10 mM each)
___ 6 µl Phusion Taq
___ Sterile H2O to 400 µl
Scalable recipe (total volume = V):
___ 1/5 V
___ 1/33 V
___ 1/40 V
___ 1/50 V
___ 1/67 V
___ 71/100 V
___Distribute 50 µl master mix per tube in an 8-well strip.
___Add 2 µl template to each well.
___Place in PCR machine. Run the following program:
98˚C 1 min, (98˚C 10 sec, 60˚C* 20 sec, 72˚C 30 sec† ) 40x, 72˚C 5 mins, 4˚C ∞ .
* Annealing temperature varies, but 60˚C works well for most primers.
† Vary elongation time as appropriate for product size.
___Run PCR products on a 2% agarose gel.
___Gel extract the PCR products, as described on page 9. Expect 10-100 ng/µl product.
___Prepare products for sequencing. Set up forward and reverse sequencing reactions for each sample.
Notes on sequencing setup:
- Begin by sequencing four putants per gene. With this many putants, there is a 99% chance of finding a frameshift, and an 80% chance of finding a frameshift into a preferred frame (see page 22), assuming an equal distribution of mutations in each frame.
- To confirm lesions, sequence two embryos carrying each of these four putations.
Analyze sequences from heterozygous DNA
___Closely examine wild-type and putant sequences. Wild-type sequence should be normal, with one prominent read per base location. Following a heterozygous indel, the wild type and mutant reads will be out of frame from one another resulting in two overlapping sequence reads (“overlapping reads”; see example on page 25).
___Align sequences against an annotated genomic wild-type reference. The overlapping reads following a frameshift should begin within the CRISPR target.
___On the aligned sequences, change 25-30 bp of the overlapping reads to ambiguous DNA coding: G/T = K A/C = M A/G = R C/T = Y C/G = S A/T = W
___In a text file, you can manually “subtract” wild-type sequences from the overlapping reads, leaving behind mutant (see example on page 25). To do this, copy/paste wild-type sequence into one line of text, and ambiguous sequence into the next. On the third line of text, generate the mutant sequence by typing the nucleotide option not found in wild-type. Align the mutant sequence to wild-type sequence to assess whether the lesion introduces a frameshifting indel.
___Alternatively, sequence separation can be done automatically, using Insilicaseʼs Heterozygous InDel software: (http://www.insilicase.co.uk/Web/HeterozygousInDels.aspx). See Notes below.
___Generate modified mRNA files carrying each lesion and pick the best alleles.
___Prioritize alleles that introduce early stop codons and minimal aberrant amino acids (see Notes below, and example on next page).
___Once an ideal lesion is identified, outcross the appropriate founder to generate a large F1 stock. In addition, raise a second frameshifting lesion to confirm mutant phenotypes.
___During these subsequent outcrosses, perform HRMA on 16 embryos at least once, to confirm that the correct founder was identified and to double-check allele frequency.
Notes on sequence analysis
- We prefer lesions where frameshift is followed by only a short stretch of aberrant amino acids before a stop codon is reached, because mutant phenotypes in these immediate-terminator alleles are easier to interpret than phenotypes in distant-terminator alleles. For instance, the addition of aberrant amino acids may have unexpected deleterious effects 17, or in exceedingly rare cases these aberrant amino acids may even cause gain of function 18, 19.
- Most alleles at a particular CRISPR target will cause frameshifts beginning within a few amino acids of one another, so minimizing the number aberrant amino acids before the stop codon usually takes precedence over finding earlier lesions.
- This analysis correctly identifies lesions from a single sequencing read. However, to reduce the possibility of errors, we sequence in both forward and reverse directions from two individuals carrying the same putation.
- The Heterozygous InDel software accurately determines mutant sequence frame, but manual inspection can usually improve the borders of alignment.
- The Heterozygous InDel software doesnʼt always provide the correct result on the first try, but errors are usually obvious. If errors occur, first check that wild-type and heterozygous sequence line up correctly. If this does not fix the output, then enter a longer stretch of sequence (>30 bp).
- The Heterozygous InDel software works well with >20 bp of overlapping reads, but shorter sequences should be manually analyzed as described above.
Example of allele selection: choosing between three six1a alleles
Three alleles were recovered from one mutagenized six1a founder (see accompanying article). All three alleles have lesions in similar locations, causing frameshift at aa 93, 93, and 94. six1aoz9 introduces the fewest aberrant amino acids (12 aa) prior to termination, and is our preferred allele for further analysis. six1aoz8 introduces 37 aberrant amino acids, and will be maintained for complementation tests. The third allele also introduces 37 aberrant amino acids, but will not be maintained because of facility space considerations and the similarity of this allele to six1aoz8.
Stock maintenance for subsequent generations
Outcross sequence confirmed F1 mutant carriers to generate F2 stocks. CRISPR mutagenesis can cause off-target mutations, though the rate of off-targeting varies considerably in different studies 13, 20. The prevalence of potential off-target mutations can be reduced by outcrossing the mutant line for several generations. After several generations of outcrossing, we recommend maintaining the line by alternating outcross generations to a wild type line (to maintain a WT background) with incrosses of heterozygous carriers (to maximize number of carriers).
Founders recovered in the F1 generation should transmit mutations to F2 progeny at standard Mendelian rates. For outcrosses, expect 50% wild-type and 50% heterozygous progeny. For incross stocks, expect 25% homozygous mutant, 50% heterozygote, and 25% homozygous wild-type progeny. If a mutation is recessive lethal, expect to find 33% wild-type and 66% heterozygous adult fish in the incross stock. Calculations of required F2 generation size, and stock sizes in subsequent generations should be based on these ratios.
VII) Confirm lesions in F2 generation homozygotes
Identify adult F1 carriers
___Clip the distal third of the caudal fin from adult F1 fish using established protocols (e.g., https://zfin.org/zf_info/zfbook/chapt7/7.8.html ). Place each fin clip in separate wells of a 96-well plate.
___Add 50 µl 1x Thermopol buffer (New England Biolabs, B9004S) to each well.
___Place in PCR machine. Run 95˚C 10 minutes, 4˚C hold.
___Add 5 µl 10 mg/ml Proteinase K to each well.
___Run 55˚C 1 hour, 95˚C 10 minutes, 4˚C hold.
___Mix samples in each well, then spin the plate to pellet the larger pieces of fin residue.
___Dilute the fin clip preparations 5-fold, by transferring 10 µl fin clip lysis to new wells containing 40 µl low TE. Mix each well containing diluted fin clip. This dilution reduces the buffer variation caused by differing sizes of fin clip, and improves HRMA consistency.
___Set up HRMA reactions as described on page 17. Be sure to include at least two wild-type fin clip controls. Expect to find the same putant curve shapes as were found during initial screening.
___Sequence confirm a few identified heterozygous fish with the ideal lesion, as described above. Use these sequence-confirmed individuals to start the next generation.
Identify F2 homozygous mutants
___Incross identified F1 adults carrying the desired lesion, after they have recovered from fin clip for at least one week.
___Lyse embryos after they have grown to at least 1 dpf, as described on page 15.
___Genotype embryos using HRMA or restriction enzyme-based genotyping. HRMA does an outstanding job of discerning heterozygotes from wild-type embryos, however homozygous wildtype DNA cannot always be distinguished from homozygous mutant DNA using HRMA (see Notes below). For this reason, a restriction enzyme-based genotyping protocol is preferred at this step.
Specific notes about using HRMA on the F2 generation
DNA homozygous for deletion alleles should usually show a curve with a lower melting temperature than wild-type DNA. DNA homozygous for insertion alleles should melt at a higher temperature than wild-type DNA.
Heterozygous DNA normalized melt curves will typically be nonparallel to both wild-type and mutant curves.
The difference between homozygous wild-type curves and homozygous mutant curves is often much subtler than the difference between either homozygote and the heterozygote. The distinctiveness of heterozygous melt curves is likely caused by heteroduplex DNA complexes, found in heterozygous PCR products.
HRMA on F2 progeny is sometimes complicated by competition in PCR reactions between wild-type and mutant products of different sizes which may dramatically alter the ratio of amplified products. This is a particular concern when the shortest HRMA PCR products (~100 bp) are used to genotype large insertions or deletions. For instance heterozygotes carrying a 14 bp deletion allele have sometimes amplified the short (WT: 99 bp, mut: 85 bp) PCR products used for HRMA as if they were mutants.
Sequence the homozygous mutation
___After genotyping F2 embryos, set up a PCR reaction for sequencing using homozygous wild-type and mutant embryo lysis for templates, as described on page 21.
___Submit PCR products for sequencing.
___Align wild-type and mutant sequences to predicted files. This time, there should be no overlapping reads, and sequence analysis uses standard methods (see example below).
___Once sequences are confirmed in F2 individuals, mutant construction is completed, and allele designation should be assigned. Each research group must obtain a unique identifier for their alleles from ZFIN (http://zfin.org/action/feature/line-designations); for instance, the Amacher lab uses “oz” alleles to designate the lines we generate at Ohio State University.
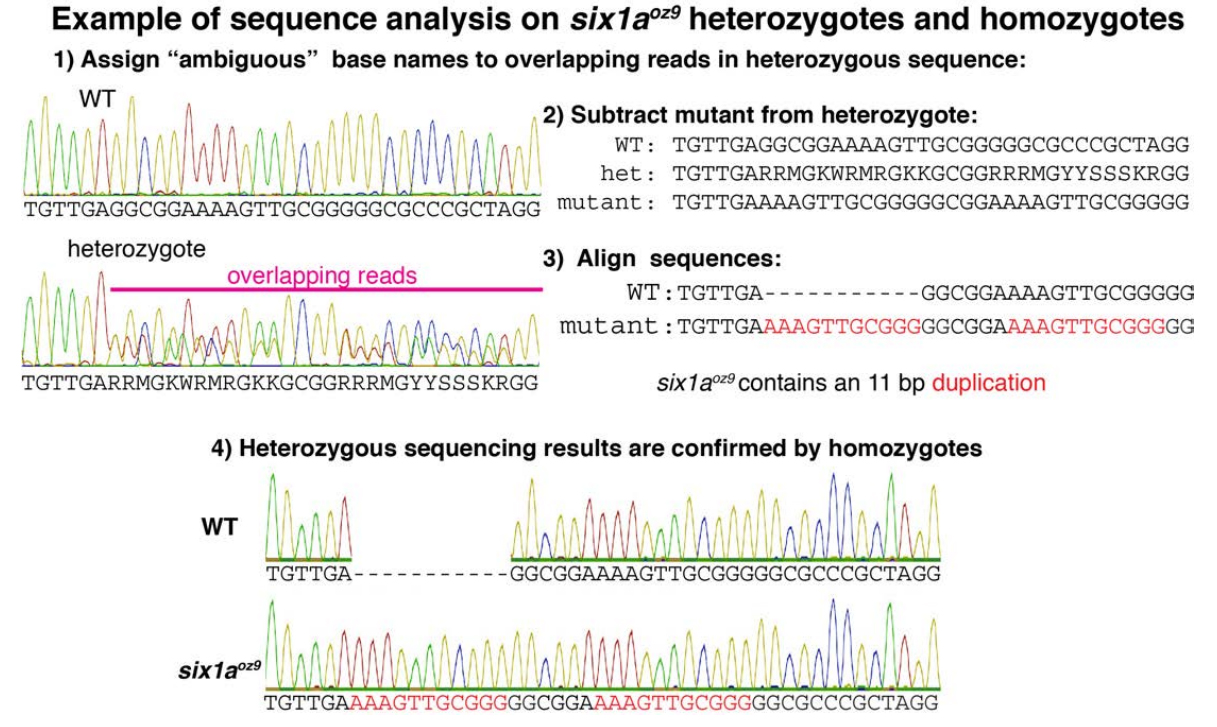
VIII) Validating the CRISPR pipeline
- CRISPR activity is sequence-specific. Previous deep sequencing analysis shows that off-target sites with 4-5 bp mismatches are mutagenized 25 to 50-fold less than the actual target site 13. To extend this analysis of specificity, we tested two CRISPRs that differ from one another at only 2 bases. We found that both effectively mutagenized their intended target, but that mutagenesis at the alternative target site was undetectable by HRMA. In our experience, it is rare to find off-target sites with less than 2 bp mismatch. However, if a CRISPR is identified with an off-target site having less than a 2 bp mismatch, we strongly recommend excluding that CRISPR during the design phase.
- Using CRISPR to a gene necessary for pigment formation (tyrosinase) 15, we find a good correlation between the degree of HRMA deflections and the degree of somatic mosaicism. For experimenters new to CRISPR mutagenesis, we recommend using a tyrosinase CRISPR as a side-by-side positive control.
- Somatic F0 CRISPR melt deflections also correlate well (R2 =0.43) with the number of putant HRMA curves transmitted through the germline. In our experience, the number of distinct putants recovered per founder can be calculated using the formula X=10.9Y, where
- X= the average deflection (in | difference-CFU | ) from the mean wild-type melt curve, assayed on F0 injected embryos
- Y= the number of distinct putant classes found per founder in F1 outcross.
- Somatic F0 CRISPR melt deflections also predict (R2 =0.47) the total rate of putants per F1 embryo. In our experience, the number of putants found per embryo can be calculated using the formula X=2.0Y, where
- X= the average deflection (in | difference-CFU | ) from the mean wild-type melt curve, assayed on CRISPR injected F0 embryos
- Y= the number of embryos in F1 outcross carrying lesions divided by the total number of embryos screened in F1 outcross.
- Putants analyzed on F1 embryos are good predictors of mutants recovered from F1 adults. For instance, all putant melt curve types identified to date from F1 embryos have also been seen when F1 adults are sequenced.
- For all lesions examined in both F1 and F2, the sequences found in F2 homozygotes have matched the mutant sequences predicted by F1 heterozygotes.
IX) Supplies and equipment
These are what weʼve tested in our lab; other versions may work too!
- CFX Connect system (Bio-Rad; 185-5201)
- qPCR: “CFX manager Software” (Bio-Rad; 185-5201)
- HRMA: “Precision Melt Analysis Software” (Bio-Rad; 184-5025)
- Ethanol (Fisher BP2818-100)
- Labnet MPS 1000 Mini Plate Spinner (Sigma; Z723533-1EA)
- LiCl, 8M solution (Sigma L7026)
- LB media and plates with 50 µg/ml Carbenicillin, 100 µg/ml Ampicillin, or 50 µg/ml Kanamycin
- MAXIscript T7 kit (Life Tech; AM1312)
- mMessage mMachine Sp6 kit (Life Tech; AM1340)
- Microseal ʻBʼ Seals (Bio-Rad; MSB1001)
- Miniprep, QiaQuick PCR cleanup kit, MinElute gel extraction kits (Qiagen; 27104, 28104, 28604)
- Qiavac 24 plus vacuum manifold (Qiagen 19413) with VacConnectors (Qiagen 19407)
- Multiplate PCR plates Low 96-well, clear (Bio-Rad; MLL9601)
- Nucleospin RNA cleanup kit (Machery Nagel; 740955.10)
- pCS2-nCas9n (https://www.addgene.org/47929/)
- pDR274 (http://www.addgene.org/42250/)
- Proteinase K (Fisher; BP1700 100)
- Precision Melt HRM Supermix (Bio-Rad; 172-5112)
- Restriction enzymes:
- BsaI-HF (New England Biolabs R3535S)
- DraI (New England Biolabs R0129S)
- NotI-HF (NEB R3189S)
- RNAse inhibitor (Promega N2515)
- T4 ligase with buffer (NEB; M0202S)
- Taq with Thermopol buffer (NEB; M0267S)
- Standard laboratory supplies for zebrafish research
X) Brief Bibliography
The following papers were invaluable for developing this protocol.
- Starck SR, Jiang V, Pavon-Eternod M, Prasad S, McCarthy B, Pan T, et al.: Leucine-tRNA initiates at CUG start codons for protein synthesis and presentation by MHC class I. Science. 2012;336:1719-23.
- Kimura K, Wakamatsu A, Suzuki Y, Ota T, Nishikawa T, Yamashita R, et al.: Diversification of transcriptional modulation: large-scale identification and characterization of putative alternative promoters of human genes. Genome Res. 2006;16:55-65.
- Dwight Z, Palais R, Wittwer CT: uMELT: prediction of high-resolution melting curves and dynamic melting profiles of PCR products in a rich web application. Bioinformatics. 2011;27:1019-20.
- Sander JD, Zaback P, Joung JK, Voytas DF, Dobbs D: Zinc Finger Targeter (ZiFiT): an engineered zinc finger/target site design tool. Nucleic Acids Res. 2007;35:W599-605.
- Sander JD, Maeder ML, Reyon D, Voytas DF, Joung JK, Dobbs D: ZiFiT (Zinc Finger Targeter): an updated zinc finger engineering tool. Nucleic Acids Res. 2010;38:W462-8.
- Bedell VM, Wang Y, Campbell JM, Poshusta TL, Starker CG, Krug RG, 2nd, et al.: In vivo genome editing using a high-efficiency TALEN system. Nature. 2012;491:114-8.
- Ran FA, Hsu PD, Wright J, Agarwala V, Scott DA, Zhang F: Genome engineering using the CRISPR- Cas9 system. Nat Protoc. 2013;8:2281-308.
- Montague TG, Cruz JM, Gagnon JA, Church GM, Valen E: CHOPCHOP: a CRISPR/Cas9 and TALEN web tool for genome editing. Nucleic Acids Res. 2014;42:W401-7.
- Hwang WY, Fu Y, Reyon D, Maeder ML, Kaini P, Sander JD, et al.: Heritable and precise zebrafish genome editing using a CRISPR-Cas system. PLoS One. 2013;8:e68708.
- Gagnon JA, Valen E, Thyme SB, Huang P, Ahkmetova L, Pauli A, et al.: Efficient mutagenesis by Cas9 protein-mediated oligonucleotide insertion and large-scale assessment of single-guide RNAs. PLoS One. 2014;9:e98186.
- Fu Y, Sander JD, Reyon D, Cascio VM, Joung JK: Improving CRISPR-Cas nuclease specificity using truncated guide RNAs. Nat Biotechnol. 2014.
- Dahlem TJ, Hoshijima K, Jurynec MJ, Gunther D, Starker CG, Locke AS, et al.: Simple methods for generating and detecting locus-specific mutations induced with TALENs in the zebrafish genome. PLoS Genet. 2012;8:e1002861.
- Hruscha A, Krawitz P, Rechenberg A, Heinrich V, Hecht J, Haass C, et al.: Efficient CRISPR/Cas9 genome editing with low off-target effects in zebrafish. Development. 2013;140:4982-7.
- Hwang WY, Fu Y, Reyon D, Maeder ML, Tsai SQ, Sander JD, et al.: Efficient genome editing in zebrafish using a CRISPR-Cas system. Nat Biotechnol. 2013;31:227-9.
- Jao LE, Wente SR, Chen W: Efficient multiplex biallelic zebrafish genome editing using a CRISPR nuclease system. Proc Natl Acad Sci U S A. 2013;110:13904-9.
- Dwight ZL, Palais R, Wittwer CT: uAnalyze: web-based high-resolution DNA melting analysis with comparison to thermodynamic predictions. IEEE/ACM Trans Comput Biol Bioinform. 2012;9:1805-11.
- Pogoda HM, Hammerschmidt M: How to make a teleost adenohypophysis: molecular pathways of pituitary development in zebrafish. Mol Cell Endocrinol. 2009;312:2-13.
- Raes J, Van de Peer Y: Functional divergence of proteins through frameshift mutations. Trends Genet. 2005;21:428-31.
- Mariano AR, Colombo E, Luzi L, Martinelli P, Volorio S, Bernard L, et al.: Cytoplasmic localization of NPM in myeloid leukemias is dictated by gain-of-function mutations that create a functional nuclear export signal. Oncogene. 2006;25:4376-80.
- Fu Y, Foden JA, Khayter C, Maeder ML, Reyon D, Joung JK, et al.: High-frequency off-target mutagenesis induced by CRISPR-Cas nucleases in human cells. Nat Biotechnol. 2013;31:822-6