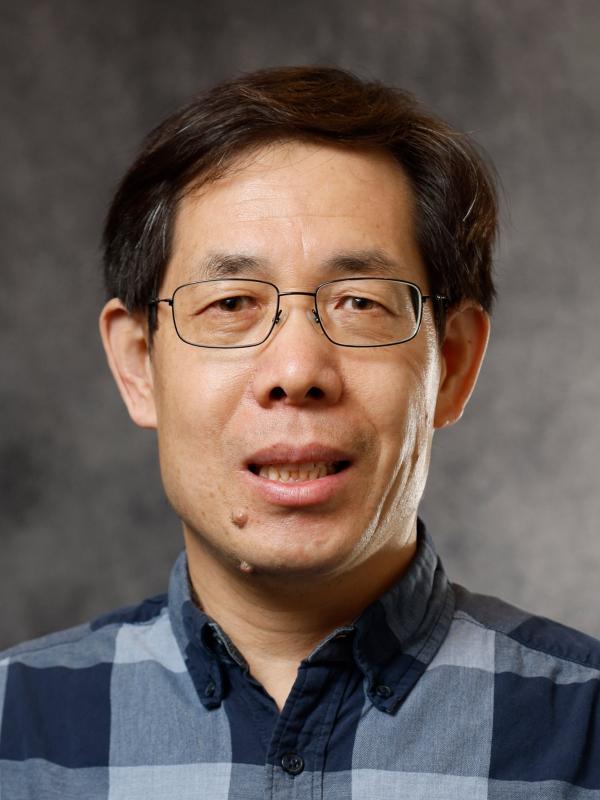
Jian-Qiu Wu
Professor
615 Biological Sciences Building
484 West 12th Avenue
Columbus, OH
43210
Areas of Expertise
- Cytokinesis
- Single-cell Wound Healing
- Cancer
Education
- Ph.D. - The University of North Carolina at Chapel Hill
- Postdoctoral - Yale University
Research Description
General Research Interests
- Cancer
- Cytokinesis
- Cellular wound healing
- Anti-fungal drug targets
Two defining characteristics of cancers are uncontrolled cell division and metastasis.
We are studying cellular processes that are related to both aspects of cancers in model systems using complementary approaches; including genetics, quantitative microscopic imaging, cell biology, biochemistry, and mathematical modeling. Cytokinesis, the final step of the cell-division cycle, is essential for cell proliferation and differentiation. Failure of cytokinesis can lead to tetraploidy, a prelude to aneuploidy and transformation of normal cells into cancer cells. Cancer cells often misregulate structural and regulatory components of cytokinesis. Since most proteins involved in cytokinesis are evolutionarily conserved, principles established in our model system of the fission yeast S. pombe can be readily applied to higher eukaryotes, including humans.
Cellular wound healing and cytokinesis are evolutionarily related processes due to both involving the actomyosin-cytoskeleton remodeling and plasma-membrane dynamics. Cellular wound healing is a ubiquitous process in eukaryotic cells and is essential for their survival. During metastasis, cancer cells are often stressed and wounded by the dense stroma and extracellular matrix. What we learn about molecular mechanisms of cellular wound healing will help us understand this intriguing biological process and design new or improved therapies to inhibit wound healing of cancer cells. Thus, our studies on cytokinesis and cellular wound healing will advance our understanding of basic fields significant to cancer research and human health.
Moreover, aspects of cytokinesis and cellular wound healing specific to fungi are excellent targets for anti-fungal drugs that have minimal impact on mammalian hosts. Approximately 1.2 billion people worldwide suffer from fungal diseases. Almost 2 million of them die of fungal infections every year. Inhibitors (caspofungin, micafungin, Aculeacin A, and others) of the conserved β-glucan synthases that build the septum for cytokinesis are used to treat fungal infections. However, anti-fungal drug development has largely stalled since the 1990s and drug resistance is rapidly emerging. Novel and basic knowledge of septum/cell wall synthesis is urgently needed. Therefore, discoveries on both conserved and organism-specific attributes of cytokinesis and cellular wound healing may be harnessed to improve human health.
Outstanding Questions in Cytokinesis
Our long-term goal is to reveal the molecular mechanisms of cytokinesis. Cytokinesis partitions cellular constituents into two daughter cells at the end of the cell cycle. From yeast to humans, cytokinesis requires the coordination of six key events: division-plane selection, actomyosin contractile-ring assembly, ring constriction and associated disassembly, targeted plasma-membrane deposition, extracellular matrix formation, and daughter-cell separation (Fig. 1). The molecular mechanisms of the last four events are least understood because the pathways overlap and the events are currently poorly resolved in time and space.
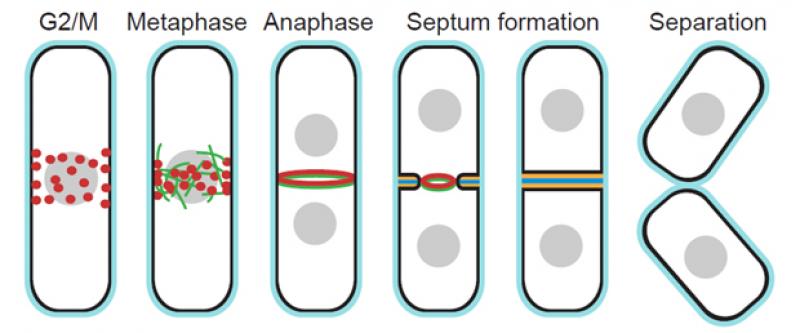
Fig. 1. Key events in fission yeast cytokinesis. Cytokinesis nodes and the actomyosin contractile ring are in red, nuclei in gray, actin filaments in green, plasma membrane in black, cell wall in light blue, primary septum in dark blue, and secondary septa in yellow.
The Fission Yeast S. pombe as a Model System to Study Cytokinesis
The fission yeast S. pombe has emerged as a favorite model system for the analysis of cytokinesis. Not only is it genetically tractable and favorable for microscopic analysis, but it also has highly efficient homologous recombination for gene targeting, a small (13.8 Mb) and fully sequenced eukaryotic genome, and perhaps most importantly, it carries out cytokinesis much like animal cells. Cytokinesis begins with the formation of nodes at the cell equator (Figs. 1 and 2). We discovered how cytokinesis nodes condense into a contractile ring by a “search, capture, pull, and release” (SCPR) mechanism (Vavylonis et al., Science 2008). Constriction of the ring is coupled with invagination of the plasma membrane and formation of a trilaminar septum, but the mechanisms coordinating these processes are unknown. Digestion of the primary septum leads to cell separation (Fig. 1). We are studying cytokinesis in S. pombe using complementary genetic, microscopic (confocal, EM, & super-resolution), biochemical, and mathematical approaches. The concepts learned from fission yeast are applicable to the coordination of actomyosin contractile structures, plasma-membrane dynamics, and extracellular matrix remodeling in animal-cell cytokinesis, cell motility, cell morphogenesis, and wound healing since most proteins are conserved.
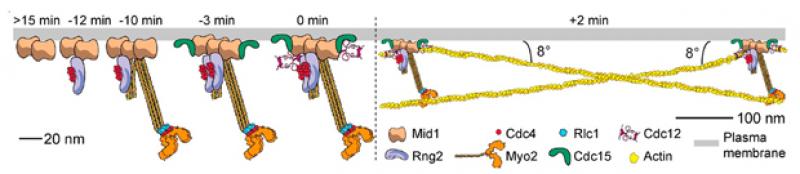
Fig. 2. Model for cytokinesis node assembly and architecture. Protein distances, stoichiometries (except Cdc12), and timings of appearance are depicted (Laporte et al., J. Cell Biol. 2011).
Current Research Projects
Project 1. Characterize the molecular mechanism of contractile-ring constriction.
Contractile-ring formation depends on actin polymerization by formins and the motor activities of myosin-II and myosin‑V (Coffman et al., J. Cell Biol. 2013; Wang et al., J. Cell Biol. 2014), but we do not know how they function during ring constriction. We will make the quantitative measurements of constricting rings required for mathematical modeling: the molecule numbers and turnover kinetics of formins, myosins, a-actinin, and cofilin, their relative positions by super-resolution microscopy, their behaviors and contractile-ring tension in mutant cells, and the biochemical activities of myosins. Then we will use these parameters to test models to account for ring constriction with 3D computer simulations. Completion of this project will elucidate the basic principles and contributions of key actin-binding proteins in contractile-ring constriction.
Project 2. Characterize the molecular mechanism that coordinates contractile-ring constriction and plasma-membrane deposition.
The prevailing model for membrane expansion during cytokinesis is that vesicles tethered by the exocyst complex insert into the plasma membrane adjacent to the contractile ring at the leading edge of the cleavage furrow. However, we have observed vesicles deposited along the whole cleavage furrow behind the constricting ring by high spatiotemporal resolution microscopy and have evidence that both the exocyst and Transport Particle Protein II (TRAPP-II) complexes participate (Wang et al., PLOS Biology, 2016). By contrast, endocytosis is more active at the rim of the cleavage furrow. In this project, we will use genetics, confocal microscopy, super-resolution microscopy, and electron tomography to determine how the balance between exocytosis and endocytosis expands the plasma membrane at the division site, determine the contributions of exocyst and TRAPP-II complexes in tethering vesicles for plasma-membrane deposition, and investigate how targeted plasma-membrane deposition coordinates with ring constriction during cytokinesis.
Project 3. Elucidate the molecular mechanism that coordinates contractile-ring constriction and septum formation.
The extracellular matrix contributes to cytokinesis in both animal cells and fungi, where the septum serves as extracellular matrix. Depletion of chondroitin proteoglycan core proteins or the secreted matrix protein hemicentin leads to cytokinesis failure in worms and mice. However, the mechanism coordinating ring constriction and extracellular matrix remodeling is poorly understood since >100 proteins are involved in these two cytokinetic stages. We hypothesize that glucan synthases delivered by vesicle trafficking coordinate ring constriction with septum formation to ensure cell integrity during cell division. We discovered three novel and conserved proteins, coiled-coil protein Rng10, glucan synthase-associated protein Sbg1, and Rng13, that may help explain the still obscure relationship between ring constriction and septum formation by regulating the localizations or stability of glucan synthases. We will characterize how the three proteins and their domains contribute to ring constriction, ring disassembly, and septum formation. Then we will elucidate how glucan synthases help anchor the contractile ring and coordinate ring constriction with septum formation. Completion of this project will reveal how glucan synthases and their regulators coordinate ring constriction and septum formation for successful cytokinesis. Conserved β- and α-glucan synthases make the glucans of the septum and cell wall in fission yeast as well as in fungal pathogens. The novel proteins and their binding partners that we discovered are conserved in fungal pathogens and thus may be ideal anti-fungal drug targets.
Project 4. Investigate how Rho GTPases regulate cytokinesis.
Rho GTPases are important regulators in all stages of cytokinesis. They are “molecular switches” that switch between the active GTP-bound and the inactive GDP-bound forms. The activity of Rho GTPases is regulated by Rho guanine nucleotide exchange factors (GEFs) and Rho GTPase activating proteins (GAPs). In human cells, the exact roles of Rho GTPases and their GEFs and GAPs in cytokinesis are still poorly understood due to the redundancies (>70 Rho GEFs in humans) and limited genetic and molecular tools in mammalian cells. We have found that Rho GEFs Rgf3, Gef2, Gef3, and RhoGAP Rga7 are involved in various stages of cytokinesis in fission yeast. Interestingly, the localizations and activities of these GEFs and GAPs are regulated by their adaptors/binding partners. We will continue investigating roles of these and other GEFs, GAPs, and their adaptors in cytokinesis.
Project 5. Elucidate the molecular mechanism of single-cell wound healing.
Single-cell (or cellular) wound healing/repair is an essential process related to cytokinesis in components and the overall pathways involved. We will use mutants or laser ablation to precisely wound specific sites at different stages of the cell cycle. Understanding the mechanism of wound healing is fundamental to biology and human health because wounds can result from diverse sources at cellular and tissue levels: environmental stress, trauma, surgery, infection, and muscle contraction. In addition, wounding can promote tumor formation and metastasis, and tumor cells could survive chemotherapy and radiation therapy using wound-healing pathways. What we learn about single-cell wound healing in fission yeast will help us understand wound responses in human cells. Therefore, new therapies could eventually be designed to facilitate wound healing, lessen the incidence of tumorigenesis, and manage tumor cells based on our conceptual breakthroughs on the molecular links between cytokinesis and single-cell wound-healing responses.
Current Research Support
- 2016-2026 An R01 grant from the National Institutes of Health
Publications
- Singh D, Liu Y, Zhu YH, Zhang S, Naegele S, Wu JQ. 2024. Septins function in exocytosis via physical interactions with the exocyst complex in fission yeast cytokinesis. eLife. Accepted. PMID: 39026698. bioRxiv [Preprint]. 2024 Jul 12:2024.07.09.602728.
- Larissa V. G. Longo, Evelyn G. Goodyear , Sha Zhang , Elena Kudryashova , Jian-Qiu Wu. 2022. Involvement of Smi1 in cell wall integrity and glucan synthase Bgs4 localization during fission yeast cytokinesis. Mol. Biol. Cell. Feb 1;33(2):ar17. PMID: 34910579.
- Gerien K.S., Zhang S., Russell A.C., Zhu Y.-H., Purde V., Wu J.-Q. 2020. Roles of Mso1 and the SM protein Sec1 in efficient vesicle fusion during fission yeast cytokinesis. Mol. Biol. Cell. 2020 May 20:mbcE20010067. doi: 10.1091/mbc.E20-01-0067. Online ahead of print. PMID: 32432970.
- Lucas B.E., McPherson M.T., Hawk T.M., Wilson L.N., Kroh J.M., Hickman K.G., Fitzgerald S.R., Disbennett W.M., Rollins P.D., Hylton H.M., Baseer M.A., Montgomery P.N., Wu J.-Q., Petreaca R.C. 2019. An Assay to Study Intra-Chromosomal Deletions in Yeast. Methods Protoc. 2(3):74. PMID: 31454903.
- Liu, Y. *, N. A. McDonald*, S.M. Naegele, K.L. Gould, and J.-Q. Wu. 2019. The F-BAR domain of Rga7 relies on a cooperative mechanism of membrane binding with a partner protein during fission yeast cytokinesis. Cell Reports. 26:2540-2548. *Co-first authors.
- Okada H. *, Wloka C. *, Wu J.-Q., Bi E. 2019. Distinct roles of myosin-II isoforms in cytokinesis under normal and stressed conditions. iScience. 14:69-87. *Co-first authors.
- Liu X.M., Yamasaki A., Du X.M., Coffman V.C., Ohsumi Y., Nakatogawa H., Wu J.-Q., Noda N.N., Du L.-L. 2018. Lipidation-independent vacuolar functions of Atg8 rely on its noncanonical interaction with a vacuole membrane protein. eLIFE. 7:e41237.
- Gerien K.S., J.-Q. Wu. 2018. Molecular mechanisms of contractile-ring constriction and membrane trafficking in cytokinesis. Biophys Rev. 10:1649-1666.
- Zhu, Y.-H., J. Hyun, Y.-Z. Pan, J.E. Hopper, J. Rizo, J.-Q. Wu. 2018. Roles of the fission yeast UNC-13/Munc13 protein Ync13 in late stages of cytokinesis. Mol. Biol. Cell. 29:2259-2279.
- Davidson R., J.A. Pontasch, J.-Q. Wu. 2016. Sbg1 is a novel regulator for the localization of the β-glucan synthase Bgs1 in fission yeast. PLoS One 11: e0167043. https://www.ncbi.nlm.nih.gov/pubmed/27898700
- Liu, Y. and J.-Q. Wu. 2016. Cytokinesis: Going super resolution in live cells. 2016. Current Biology. 26:R1150-1152.
- Liu, Y., I-J. Lee, M. Sun, C.A. Lower, K.W. Runge, J. Ma, and J.-Q. Wu. 2016. Roles of the novel coiled-coil protein Rng10 in septum formation during fission yeast cytokinesis. Mol. Biol. Cell. 27:2528-2541.
- Davidson R., Y. Liu, K.S. Gerien, and J.-Q. Wu*. 2016. Real-time visualization and quantification of contractile ring proteins in single living cells. Methods in Mol. Biol. 1369:9-23
- Wang, N., I-J. Lee, G. Rask, and J.-Q. Wu*. 2016. Roles of the TRAPP-II Complex and the Exocyst in Membrane Deposition during Fission Yeast Cytokinesis. PLoS Biology. 14:e1002437.
- Sun, L., R. Guan, I-J. Lee, Y. Liu, M. Chen, J. Wang, J.-Q. Wu, Z. Chen. 2015. Mechanistic insights into the anchorage of the contractile ring by anillin and Mid1. Developmental Cell. 33:413-426.
- Xu, T., D. Vavylonis, F.-C. Tsai, G. Koenderink, W. Nie, E. Yusuf, I-J. Lee, J.-Q. Wu, and X. Huang. 2015. SOAX: A software for quantification of 3D biopolymer networks. Scientific Reports. 5:9081.
- Davidson, R., D. Laporte, and J.-Q. Wu*. 2015. Regulation of Rho GEF Rgf3 by the arrestin Art1 in fission yeast cytokinesis. Mol. Biol. Cell. 26:453-466.
- Wang, N., M. Wang, Y.-H. Zhu, T.W. Grosel, D. Sun, D.S. Kudryashov, and J.-Q. Wu*. 2015. The Rho-GEF Gef3 interacts with the septin complex and activates the GTPase Rho4 during fission yeast cytokinesis. Mol. Biol. Cell. 26:238-255.
- Coffman, V.C., I-J. Lee, and J.-Q. Wu*. 2014. Counting molecules within cells. Colloquium Series on Quantitative Cell Biology (e-book series). Biota Publishing, ISBN# 9781615046553.
- Wang, N., L. Lo Presti, Y.-H. Zhu, M. Kang, Z. Wu, S.G. Martin, and J.-Q. Wu*. 2014. The novel proteins Rng8 and Rng9 regulate the myosin-V Myo51 during fission yeast cytokinesis. J. Cell Biol. 205:357-375.
- [Highlighted by JCB In Focus at http://jcb.rupress.org/content/205/3/284.2.full].
- Lee, I.-J., N. Wang, W. Hu, K. Schott, J. Bähler, T.H. Giddings, Jr., J.R. Pringle, L.-L. Du, J.-Q. Wu*. 2014. Regulation of spindle-pole body assembly and cytokinesis by the centrin-binding protein Sfi1 in fission yeast. Mol. Biol. Cell. 2014 Jul 16. pii: mbc.E13-11-0699. [Epub ahead of print].
- Deng, L, R. Kabeche, N. Wang, J.-Q. Wu, J.B. Moseley. 2014. Megadalton node assembly by binding of Skb1 to the membrane anchor Slf1. Mol. Biol. Cell. 2014 Jul 9. pii: mbc.E14-04-0896. [Epub ahead of print].
- Coffman, V.C*. and J.-Q. Wu*. 2014. Every laboratory with a fluorescence microscope should consider counting molecules. Mol. Biol. Cell. 25:1545-1548. Review.
- Zhu, Y.-H. and J.-Q. Wu*. 2014. Cell-size control – complicated. Cell Cycle. 13:693-694.
- Coffman V.C., J.A. Sees, D.R. Kovar, and J.-Q. Wu*. 2013. The formins Cdc12 and For3 cooperate during contractile ring assembly in cytokinesis. J. Cell Biol. 203:101-114.
- Zhu Y.-H., Y.F. Ye, Z. Wu, and J.-Q. Wu*. 2013. Cooperation between Rho-GEF Gef2 and its binding partner Nod1 in the regulation of fission yeast cytokinesis. Mol. Biol. Cell. 24:3187-3204.
- Coffman, V.C. and J.-Q. Wu. 2012. Counting protein molecules using quantitative fluorescence microscopy. Trends in Biochemical Sciences. 37:499-506.
- Lee, I-J., V.C. Coffman and J.-Q. Wu. 2012. Contractile-ring assembly in fission yeast cytokinesis: recent advances and new perspectives. Cytoskeleton. 69:751-763.
- Laporte, D., N. Ojkic, D. Vavylonis, J.-Q. Wu. 2012. Alpha-Actinin and fimbrin cooperate with myosin II to organize actomyosin bundles during contractile-ring assembly.Mol. Biol. Cell. 23:3094-3110.
- Lee, I-J. and J.-Q. Wu. 2012. Characterization of Mid1 domains for targeting and scaffolding in fission yeast cytokinesis. J. Cell Sci. 125:2973-2985.
- Y.F. Ye, I-J. Lee, K.W. Runge, and J.-Q. Wu*. 2012. Roles of putative Rho-GEF Gef2 in division-site positioning and contractile-ring function in fission yeast cytokinesis. Mol. Biol. Cell. 23:1181-1195.
- Valerie C. Coffman, P. Wu, M. Parthun, and J.-Q. Wu. 2011. CENP-A Exceeds Microtubule Attachment Sites in Centromere Clusters of both Budding and Fission Yeast. J. Cell Biology 195:563-572. [Highlighted by JCB In Focus at: http://jcb.rupress.org/content/195/4/539.full].
- P. Wu, R. Zhao, Y. Ye, and J.-Q. Wu. 2011. Roles of the DYRK Kinase Pom2 in Cytokinesis, Mitochondrial Morphology, and Sporulation in Fission Yeast. PLoS ONE. 6(12):e28000.
- Ojkic N., J.-Q. Wu., Vavylonis D. 2011. Model of myosin node aggregation into a contractile ring: the effect of local alignment. J. Phys Condensed Matter 2011 Sep 21;23(37):374103. Epub 2011 Aug 23.
- Laporte, D., V.C. Coffman, I-J. Lee, and J.-Q. Wu. 2011. Assembly and architecture of precursor nodes during fission yeast cytokinesis. J. Cell Biol. 192: 1005-1021. [Highlighted by JCB at: http://jcb.rupress.org/content/192/6/902.2.full].
- Pollard, T.D. and J.-Q. Wu. 2010. Understanding cytokinesis: lessons from fission yeast. Nature Reviews Molecular Cell Biology. 11: 149-155.
- Laporte, D., R. Zhao, and J.-Q. Wu. 2010. Mechanisms of contractile-ring assembly in fission yeast and beyond. Seminars in Cell & Developmental Biology. http://www.ncbi.nlm.nih.gov/pubmed/20708088
- Wu, J.-Q., Y.F. Ye, N. Wang, T.D. Pollard, and J.R. Pringle. 2010. Cooperation between the septins and the actomyosin ring and role of a cell-integrity pathway during cell division in fission yeast. Genetics. http://www.ncbi.nlm.nih.gov/pubmed/20739711
- Onishi, M., T. Koga, A. Hirata, T. Nakamura, H. Asakawa, C. Shimoda, J. Bähler, J.-Q. Wu, K. Takegawa, H. Tachikawa, J.R. Pringle, Y. Fukui. 2010. Role of septins in the orientation of forespore-membrane extension during sporulation in fission yeast. Mol. Cell Biol. 30: 2057-2074.
- Coffman, V.C., A.H. Nile, I-J. Lee, H. Liu, and J.-Q. Wu. 2009. Roles of formin nodes and myosin motor activity in Mid1p-dependent contractile ring assembly during fission yeast cytokinesis. Mol. Biol. Cell. 20: 5195-5210. [Highlighted in MBC InCytes, ASCB Newsletter, will be considered for MBC Paper of the Year.]
- Vavylonis*, D, J.-Q. Wu*, S. Hao, B. O'Shaughnesy and T.D. Pollard. (2008). Assembly Mechanism of the Contractile Ring for Cytokinesis by Fission Yeast. Science 319: 97-100. *Co-first authors.
- Wu, J.-Q., C.D. McCormick, and T.D. Pollard. 2008. Counting proteins in living cells by quantitative fluorescence microscopy with internal standards. Methods in Cell Biol. 89: 253-273.
- Wu, J.-Q., V. Sirotkin, D.K. Kovar, M. Lord, C.C. Beltzner, J.R. Kuhn, and T.D. Pollard. (2006). Assembly of the cytokinetic contractile ring from a broad band of nodes in fission yeast. J. Cell Biol. 174: 391-402.
- Kovar, D.R., J.-Q. Wu, and T.D. Pollard. (2005). Profilin-mediated competition between capping protein and formin Cdc12p during cytokinesis in fission yeast. Mol. Biol. Cell 16: 2313-2324.
- Wu, J.-Q. and T.D. Pollard. 2005. Counting cytokinesis proteins globally and locally in fission yeast. Science 310: 310-314.
- Wu, J.-Q., J.R. Kuhn, D.R. Kovar, and T.D. Pollard. (2003). Spatial and temporal pathway for assembly and constriction of the contractile ring in fission yeast cytokinesis. Dev. Cell 5: 723-734.
- Wu, J.-Q., J. Bähler, and J.R. Pringle. (2001). Roles of a fimbrin and an a-actinin-like protein in fission yeast cell polarization and cytokinesis. Mol. Biol. Cell 12: 1061-1077.
- Hales, K.G., E. Bi, J.-Q. Wu, J.C. Adam, I.-C. Yu, and J.R. Pringle. (1999). Cytokinesis: an emerging unified theory for eukaryotes? Curr. Opin. Cell Biol. 11: 717-725.
- Bähler, J.*, J.-Q. Wu*, M.S. Longtine, N.G. Shah, A. McKenzie III, A.B. Steever, A. Wach, P. Philippsen, and J.R. Pringle. (1998). Heterologous modules for efficient and versatile gene targeting in Schizosaccharomyces pombe. Yeast 14: 943-951. *Co-first authors.